Review Article | DOI: https://doi.org/10.31579/2835-7957/008
The Role of Hypoxia-Induced Factor in Cell Metabolism
1 Candidate of biological science, assistant professor of pathophysiology department, Grodno State Medical University, Gorky St., Grodno, Belarus.
2 Grodno State Medical University, Gorky St., Grodno, Belarus.
*Corresponding Author: Dremza Bon L.I, Candidate of biological science, assistant professor of pathophysiology department, Grodno State Medical University, Gorky St., Grodno, Belarus.
Citation: Maksimovich N. Ye., Dremza I. K. Bon L.I., (2023). The Role of Hypoxia-Induced Factor in Cell Metabolism. Clinical Reviews and Case Reports.2(1); DOI:10.31579/2835-7957/008
Copyright: © 2023, Dremza Bon L.I. This is an open-access article distributed under the terms of the Creative Commons Attribution License, which permits unrestricted use, distribution, and reproduction in any medium, provided the original author and source are credited.
Received: 06 January 2023 | Accepted: 17 January 2023 | Published: 26 January 2023
Keywords: hypoxia-induced factor; hypoxia; metabolism
Abstract
Objective: Analysis and synthesis of literature data on morphological and functional properties and the diagnostic value of induced factor hypoxia.
Methods: The basis of this study was a review of literature on this topic.
Results: An important role in the adaptation of the organism to hypoxia belongs to a specific regulatory protein - hypoxia-induced factor (HIF), the activity of which increases with decreasing oxygen tension in the blood. HIF is a heterodimeric protein, the beta subunit of which is constantly expressed, and the synthesis of the alpha subunit is regulated by oxygen.
Conclusion: Acute oxygen deficiency is the basis of a variety of pathological processes in many diseases and environmental factors. The hypoxia-induced factor is responsible for the formation of long-term adaptation to hypoxia, and therefore is a suitable target for pharmacological effects. The search for drugs that act as inducers or inhibitors of its synthesis is an important area in experimental pharmacology, since it allows not only to regulate the processes of adaptation to hypoxia, but more effectively treat cerebrovascular, cardiovascular, oncological and other diseases in whose genesis the leading role plays oxygen deficiency.
Introduction
The issues of increasing the body's resistance to oxygen deficiency and energy deficiency are relevant, since hypoxia, as a typical pathological process, in one way or another initiates the development and accompanies the course of all types of pathology, leading to functional and then structural changes in organs and tissues as a result of a decrease in intracellular oxygen tension [7,22].
In recent years, the fundamental mechanisms of the development of hypoxia of various genesis and the metabolic and functional disorders induced by it at the level of cells and subcellular structures have been studied. A number of morpho functional structures have been identified that are directly involved in the development of urgent and long-term adaptation of the body to hypoxia. These structures can act as specific targets for the effects of pharmacological agents in order to regulate the processes of adaptation of the body to hypoxia, which opens up promising opportunities for the search and development of new effective drugs with ant hypoxic action [3,7].
An important role in the adaptation of the body to hypoxia belongs to a specific regulatory protein – hypoxia-induced factor (HIF), whose activity increases with a decrease in oxygen tension in the blood. Hypoxia–induced factors are a group of transcription factors that respond to a decrease in oxygen content in cells or to hypoxia (Table 1) [3].
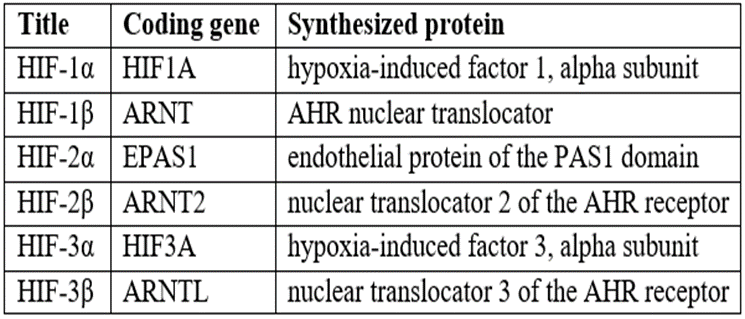
It has been shown that HIF plays a major role in the systemic response of the body to hypoxia, is synthesized in many tissues of the body, including in nervous tissue, where its expression is maximal in neurons. HIF-1 functions as the main transcription regulator of the adaptive response to hypoxia. When it is expressed, more than 40 genes are activated, whose protein products increase oxygen delivery or promote metabolic adaptation to hypoxia [2].
A highly conserved HIF-1 transcription complex belonging to the PER-ARNT-SIM (PAS) subfamily of the basic family of transcription factors is expressed in humans and animals [6].
In the presence of oxygen (normoxia), prolyl hydroxylase (PHD) hydroxylates HIF-1α, which as a result binds to the pHVL adaptive protein, is ubiquitinated and destroyed in the S26 proteasome. In hypoxia, prolyl hydroxylase loses activity, and HIF-1α dimerizes in the cell nucleus with permanently active HIF-1β, thus activating transcription of a number of genes that ensure cell adaptation to hypoxia. With a significant deficiency of ATP, the cell may undergo apoptosis (along the mitochondrial pathway), and in case of inefficiency of adaptation mechanisms, necrosis [14, 15, 16]
HIF-1 is a heterodimeric protein, the beta subunit of which is constantly expressed, and the synthesis of the alpha subunit is regulated by oxygen. HIF-1 subunits contain three domains: the N-terminus is the bHLH domain for DNA binding, the central region is the Per–ARNT–Sim (PAS) domain, which facilitates heterodimerization, and the C-terminus, responsible for the spatial orientation of transcriptional coregulatory proteins [4].
At normal oxygen concentration, the amino acid residues of proline of the HIF-1 alpha molecule are hydroxylated as a result of the activity of the O2 and/or Fe-dependent enzyme prolyl hydroxylase, which is a molecular oxygen sensor. The modified HIF-1 alpha subunit undergoes proteasome degradation through a number of stages. In a state of hypoxia, the HIF-1 alpha protein molecule does not hydroxylate, remains stable and accumulates. HIF-1 alpha and HIF-1 beta subunits are combined. The resulting HIF-1 transcription protein in the cell nucleus binds to special DNA sequences in genes whose expression is induced by hypoxia [5].
HIF-1 synthesis can be realized through oxygen-independent mechanisms. Thus, HIF-1 is synthesized in reactions controlled by signaling systems such as MAPK (mitogen activated proteinkinase – activated by signals that promote proliferation) and PI3K (phosphatidylinositol-3 kinase – a regulatory protein located at the intersection of various signaling pathways and controlling key cell functions). Of particular importance in the regulation of such functions as growth, survival, aging, tumor transformation. It should be noted that PI3K belongs to a group of enzymes united under the name "kinases that save from reperfusion damage" (RISK) [7]. These kinases are believed to act as targets for pharmacological effects in reperfusion injuries. Activation of this group of enzymes leads to inhibition of the opening of mitochondrial pores, as a result of which a cytoprotective effect is realized. The MAPK and PI3K signaling systems are activated through the tyrosine kinase receptor, the specific succinate-dependent receptor GPR-91, etc. Receptor agonists are tyrosine hydroxylase, cytokines, growth factors (for example, insulin-like growth factor), succinate [12,18].
An increase in the level of HIF-1 leads to an increase in the expression of genes that ensure cell adaptation to hypoxia and stimulate erythropoiesis (erythropoietin genes), angiogenesis (vascular endothelial growth factor gene), glycolysis enzymes (aldolase gene, lactate dehydrogenase, phosphofructokinase, pyruvate kinase, etc.). In addition, HIF-1 regulates the expression of genes involved in iron metabolism, regulation of vascular tone, cell proliferation, apoptosis, lipogenesis, formation of carotid glomeruli, development of B-lymphocytes, etc.
It has been shown that the protein antagonizing muscle A-kinase (mAKAP), by stimulating ubiquitin-E3 ligase, affects the stability and positioning of HIF-1 in the nucleus. Depletion of mAKAP or disruption of its effect on the perinuclear region in cardiomyocytes alters the stability of HIF-1 and transcriptional activation of genes associated with hypoxia. Thus, the "compartmentalization" of oxygen-sensitive signaling components can affect sensitivity to hypoxia [1,14,19].
HIF synthesis is regulated by other transcription factors involved in protecting the cell from damage. Transcription factor NF-kB (nuclear factor "kappa-bi") is a universal transcription factor that controls the expression of immune response, apoptosis and cell cycle genes, and is a direct modulator of HIF–1 expression under normoxia conditions [1,19].
Regulation of the HIF-1 α-subunit is carried out by two enzymatic reactions in which molecular oxygen reacts with two specific amino acid residues: in the presence of oxygen, the prolyl residue is hydroxylated in the oxygen-dependent region and oxygen is attached to the aspartic residue near the C-terminal region of HIF-1. Both reactions are catalyzed by 2-oxoglutarate dependent dioxygenases, which belong to a family of enzymes with diverse biological functions. These enzymes belong to prolyl hydroxylase proteins and are identified as prolyl hydroxylation catalases (PHD) HIF. The HIF-1 modified in this way binds to the tumor-suppressor protein VHL gene, which has ubiquitin ligase activity, which leads to the degradation of HIF-1 α. At low oxygen levels, HIF-1 α forms an active complex with a β-subunit, as a result of which it becomes stable and combines with cytochrome P300 [10,11,20].
In addition to the main modification of HIF, there is also the modulation of this protein by phosphorylation and acetylation. In addition, increased expression of hydroxylase genes are observed in hypoxic cells, which leads to inhibition of HIF proteins by feedback. In the presence of oxygen, the enzyme FIH (HIF inhibiting factor) also acts, which inhibits the transcriptional activity of HIF-1 [8, 9, 17, 23].
Tumor-suppressor protein VHL, consisting of 213 amino acid residues, plays a critical role in the regulation of HIF-1, since it is responsible for ubiquitization of HIF-α subunits in the presence of oxygen. In this process, VHL acts as a recognition component of the ubiquitin ligase complex. Experiments on cell cultures have shown that VHL is necessary for the regulation of HIF destruction in cells of all types. After hydroxylation, HIF-1α binds to VHL, after which it is ubiquitalized and then destroyed by proteases.In cells where VHL is insufficient, HIF-1a chains remain active even at normal oxygen partial pressure [7].
In studies on cell culture, it has been shown that in cases when the prolyl hydroxylation of HIF-α is inactivated and, accordingly, degradation is inhibited, stabilization and accumulation of α-chains of this protein occur. Then HIF-α moves to the nucleus, where it dimerizes with HIF-β, binds to hypoxia by the responsible elements of HIF genes, activating their transcription. At normal VHL levels, the C-terminal aspartic residue is hydroxylated by enzymes of the FIH group, which leads to a decrease in the transcriptional activity of the HIF complex. Therefore, it is believed that the VHL – HIF chain can be considered a central regulator of oxygen homeostasis [8].
Inactivation of HIF-1α, HIF-2α, HIF-β or VHL in mice during embryogenesis leads to the death of the embryo in utero or in the perinatal period. Mice homozygous for HIF-1a deficiency die in utero between the 8th and 11th days due to neural tube defect or cardiovascular deformities. In mice with HIF-2a deficiency, changes in the synthesis of catecholamine are observed, leading to heart damage, and violations in the synthesis of VEGF cause damage to the lungs, yolk protein, accompanied by mitochondrial abnormalities [24]. Mice with HIF-1ß deficiency die from pronounced disorders of the vasculogenesis of the yolk sac and bronchi. Inactivation of VHL causes an increase in the transcriptional activity of HIF-1a and HIF-2a, therefore, mice with a deficiency of this factor die during gestation due will to abnormal placental vasculogenesis. These studies show the importance of the HIF system for fetal development [1-4].
Under normal physiological conditions in adult mammals, maintaining the HIFI system at a certain level in all organs and tissues is also extremely important, especially for kidney tissue. HIF-α subunits are determined in kidney cells — in the cortical and medular layers, in S-corpuscles and glomerular cells. In the regulation of erythropoiesis, the kidneys play a very important role, since they serve as the main physiological oxygen sensor, responding to systemic hypoxia with a rapid increase in the production of erythropoietin (EPO) in renal interstitial cells. The liver is also involved in the production of EPO, but in a much smaller amount than the kidneys, and if EPO production in the kidneys is disrupted, its extrarenal synthesis cannot compensate for renal losses [15].
Naturally, the main regulator of EPO production is HIF-1a, which was discovered during the study of EPO regulation. However, it has now been shown that HIF-2a also participates in the regulation of erythropoiesis in both the liver and kidneys, but its formation is more pronounced in the liver. However, it has now been shown that HIF-2a also participates in the regulation of erythropoiesis in both the liver and kidneys, but its formation is more pronounced in the liver [20,23].
HIF and iron are known to interact via iron-regulated proteins (IRP's): IRP-1 and IRP-2. IRP's post-transcriptionally regulate the expression of iron metabolism proteins by binding them to the matrix RNA (mRNA) of iron–regulated elements (IRE's). When the iron reserves in the cell are depleted, the IRP-IRE complex prevents sequestration of the transferrin receptor and thereby enhances the capture of iron by the cell; with sufficient iron in the cell, the IRP-IRE complex is inactivated, undergoes protosomal degradation and iron is not absorbed. It is important to note that PHD is included in the degradation pathway of this complex – the same enzyme as in the hydroxylation of HIF [11]. In addition, it was found that HIF-2a is also posttranslationally regulated by the IRP-IRE complex. It is shown that in iron deficiency HIF-2a is included in the control mechanism according to the feedback principle to limit the production of EPO in order to prevent the development of even deeper iron deficiency [10].
HIF also plays an important role in physiological processes at the level of whole organs: during lung ventilation, in the work of the heart, etc. Hypoxia can be caused by rising to a great height, which is accompanied by significant changes in the level of physiological reactions and is accompanied by HIF activation [12].
The HIF factor is responsible for the formation of long-term adaptation to hypoxia, and therefore is a suitable target for pharmacological effects. The search for medicinal substances acting as inducers or inhibitors of its synthesis is an urgent direction in experimental pharmacology, since it allows not only to regulate the processes of adaptation to hypoxia, but also to treat cerebrovascular, cardiovascular, oncological and other diseases more effectively, in the genesis of which oxygen deficiency plays a leading role.
References
- Ang S., Chen H., Gordeuk V. (2002). Endemic polycythemia in Russia: mutation in the VHL gene, Blood Cells, Molecules and Disease.N28. P. 57-62.
View at Publisher | View at Google Scholar - Appelhoff R., Tian Y., Raval R. (2004). Differential function of the prolyl hydroxylases PHD 1, PHD 2 and PHD 3 in the regulation of hypoxia-inducible factor, J. Biol. Chem. N279. P. 38458-38465.
View at Publisher | View at Google Scholar - Benizri E., Ginouvès A., Berra E. (2008). The magic of the hypoxia-signaling cascade, Cellular and Molecular Life Sciences. N65. P. 1133-1149.
View at Publisher | View at Google Scholar - Bosco M., D'Orazi G., Del Bufalo D. (2020). Targeting hypoxia in tumor: a new promising therapeutic strategy, J Exp Clin Cancer Res. N10. P. 1-8.
View at Publisher | View at Google Scholar - Chen X., Yao J.M., Fang X., Zhang C., Yang Y., Hu C.P., (2019). Hypoxia promotes pulmonary vascular remodeling via HIF-1α to regulate mitochondrial dynamics, J Geriatr Cardiol. N16. P. 855-871.
View at Publisher | View at Google Scholar - Formenti F., Constantin-Teodosiu D., Emmanuel Y., Cheeseman J., Dorrington K., et al. (2010). Regulation of human metabolism by hypoxia-inducible factor, Proceedings of the National Academy of Sciences of the United States of America. N107. P. 2722-12727.
View at Publisher | View at Google Scholar - Gleadle J., Rateliffe P., Pugh C. (2002). Hypoxia-inducible factor (HIF) asparagine hydrolase is identical to factor inhibiting HIF (FIH) and is related to the cup in structural family, Biol. Chem. N277. P. 26351-26355.
View at Publisher | View at Google Scholar - Haase V. (2010). Hypoxic regulation of erythropoiesis and iron metabolism, American Physiological Society. N1. P. 1-13.
View at Publisher | View at Google Scholar - Haase V. (2006). Hypoxia-inducible factors in the kidney, Am J Physio Renal Physiol. N291. P. 271-281.
View at Publisher | View at Google Scholar - Haase V. (2005). The VHL tumor suppressor in development and disease: functional studies in mise by conditional gene targeting, Semin. Cell Dev. Biol. N16. P. 564-574.
View at Publisher | View at Google Scholar - Hirota K., Semenza G. (2006). Regulation of angiogenesis by hypoxia-inducible factor 1, Critical Reviews in oncology/hematology. N59. P. 15-26.
View at Publisher | View at Google Scholar - Hu C., Jyer S., Sataur A. (2006). Differential regulation of the transcriptional activities of hypoxia-inducible factor 1a (HIF 1a) and HIF-2a in stem cells, Molec. and Cell Biol. N26. P. 3514-3526.
View at Publisher | View at Google Scholar - Maxwell P. (2003). HIF-1: An oxygen response system with special relevance to the kidney, J. Am. Soc. Nephrol. N14. P. 2712-2722.
View at Publisher | View at Google Scholar - Min J., Yang H., Ivan M., Gertler F., Kaelin W., et al. (2002). Structure of an HIF-1alpha-pVHL complex: hydroxyproline recognition in signaling, Science. N5574. P. 1886-1889.
View at Publisher | View at Google Scholar - Pezzuto A, Perrone G, Orlando N, Citarella F, Ciccozzi M, et al. (2019). A close relationship between HIF-1α expression and bone metastases in advanced NSCLC, a retrospective analysis, Oncotarget. N10. P. 7071-7079.
View at Publisher | View at Google Scholar - Smith T., Robbins P., Ratcliffe P. (2008). The human side of hypoxia-inducible factor, British Journal of Haematology. N3. P. 325-334.
View at Publisher | View at Google Scholar - Triantafyllou E. Mylonis I., Simos G., Paraskeva E. (2019). Hypoxia Induces Pro-Fibrotic and Fibrosis Marker Genes in Hepatocellular Carcinoma Cells Independently of Inflammatory Stimulation and the NF-κΒ Pathway, Hypoxia. N7. P. 87-91.
View at Publisher | View at Google Scholar - van Uden P., Kenneth N., Rocha S. (2008). Regulation of hypoxia-inducible factor-1alpha by NF-kappaB, The Biochemical Journal. N412. P. 477-484.
View at Publisher | View at Google Scholar - Waqas M., Qamar H., Zhang J., Yao W., Li A., et al. (2020). Puerarin enhance vascular proliferation and halt apoptosis in thiram-induced avian tibial dyschondroplasia by regulating HIF-1α, TIMP-3 and BCL-2 expressions, Ecotoxicol Environ Saf. N6. P. 190-198.
View at Publisher | View at Google Scholar - Wilkins S., Abboud M., Hancock R., Schofield C. (2016). Targeting Protein-Protein Interactions in the HIF System, Med Chem. N8. P. 773-786.
View at Publisher | View at Google Scholar - Wong W., Goehring A., Kapiloff M., Langeberg L., Scott J. D. (2008). mAKAP compartmentalizes oxygen-dependent control of HIF-1alpha, Science Signaling. 2008. N51. P. 18-23.
View at Publisher | View at Google Scholar - Wu D., Cao W., Xiang D., Hu Y., Luo B., et al. (2020). Exercise induces tissue hypoxia and HIF-1α redistribution in the small intestine, J Sport Health Sci. N9. P. 82-89.
View at Publisher | View at Google Scholar - Yang X., Zeng M., Shao L., Jiang G., Cheng J., et al. (2019). NFAT5 and HIF-1α Coordinate to Regulate NKCC1 Expression in Hippocampal Neurons After Hypoxia-Ischemia, Front Cell Dev Biol. N7. P. 339-342.
View at Publisher | View at Google Scholar - Yoon D., Pastore Y., Divoky V. (2006). Hypoxia-inducible factor 1 deficience results in dysregulated erythropoiesis signaling and iron homeostasis in mouse development, J. Biol. Chem. N281. P. 25703-25711.
View at Publisher | View at Google Scholar